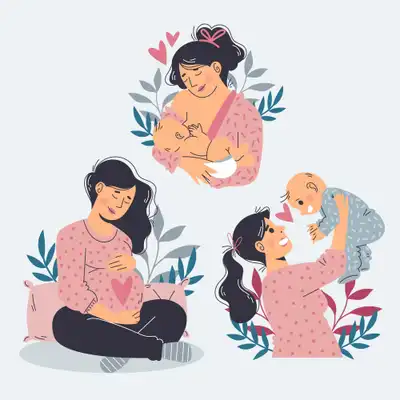
Your skin during pregnancy
How does the skin seamlessly stretch and expand itself around a growing foetus as pregnancy progresses? The answer lies in the skin proteins - collagen and elastin.
The human body, on an average, has about 37.2 trillion cells (Bianconi et al., 2013). Each cell, as minuscule as it is, is a highly efficient system with thousands of molecules diligently regulating its affairs. It is the sincere coordination between these cells that keeps our body functioning and alive. But how did this highly evolved unit come into being and what is it made of? We see thousands of living organisms around us. Are all those organisms made of the same kind of cell, or are they all different? If we were to zoom into a single cell in our body, what would we find inside it? Are there ways to study what goes on inside the cell? To find answers to these questions, let’s take a peep into the story of the cell.
Here I recreate using acrylic art, Robert Hooke’s depiction of cells as observed under the microscope. © Sunaina Rao.
In the 1600’s, a scientist named Robert Hooke who was working with the Royal Society in London, was asked to give periodic microscopic demonstrations. To this end, he started to observe a huge array of objects. He observed cloth fibres, insects, crystals and the surface of the moon, under a microscope and a telescope and then made detailed scientific illustrations of the same. He published several of such illustrations in his work Micrographia (Hooke and undefined, 2011) and one such illustration in this book was that of what he observed while looking at a piece of plant material. What he saw were repetitive units, which reminded him of the cells in a monastery and he hence called the unit as a ‘cell’ owing to its appearance. Little did he know back then that he had indeed looked at the building units of the plant and had opened the eyes of humanity to a so far unknown world.
After decades of studies and experimentation, scientists now know that it took millions of years of gradual evolution for cells to form (Wacey et al., 2011). We know by the huge amounts of microscopic data (Schopf et al., 2007) available, that all cells are composed of some very specific macromolecules, which are like beads strung on a chain. So, to make this chain (the macromolecule) we first need its individual beads (subunits). The most fundamental macromolecules that make all cells are:
So, if we were to engineer a cell, we would need all these macromolecules and hence their respective subunits:
Here I depict using acrylic art, primitive cells containing some form of genetic material (blue) enclosed within a lipid layer (yellow). © Sunaina Rao.
What this says is that the macromolecules that make the cell, must have started (Wacey et al., 2011) to form much before that.
Several theories have been put forth, explaining how cells started to form on primitive earth. An experiment that really stood out was that conducted by Stanley Miller, published in the year 19535. It was believed that the primitive earth had an oxygen free environment, with predominant gases like methane, ammonia and hydrogen along with lots of water and solar energy. So, Miller built an apparatus that could circulate these gases and water past an electric discharge. The resultant mixture was found to contain several amino acids (remember, the subunits that form proteins) along with other organic molecules. Although the setting of this experiment has some strong opposition, what it proved anyway was that organic molecules can be synthesised from inorganic gases, and this could have been a possibility, if not an absolute reality.
These organic molecules could have then bonded together to form the larger molecules under the right conditions. Some lipid molecules could have then formed a bubble-like structure (owing to their chemical nature) trapping some kind of genetic material (DNA/RNA) within it.
For anything to be considered a life, it should be able to make more of itself (reproduce). So, for this bubble to be ‘alive’, it needs a genetic material that can copy itself and make more such bubbles with the same genetic material. In all the current life forms, DNA (Deoxyribonucleic acid) is the predominant genetic material and RNA (Ribonucleic acid) helps translate the genetic information in the DNA into specific proteins, which are essentially the workers of the cell. But there is lot of debate on which could have been the first genetic material – the DNA or RNA. The two molecules are very similar in structure, except that DNA is usually double stranded, and RNA single stranded, amongst other minor structural variations. Based on what we know of DNA presently, it requires specific proteins to make copies of, or replicate itself, and cannot work independently. But RNA on the other hand, has been found to have the ability to make and break bonds between nucleotides and hence could have very well replicated itself. This is the underlying principle of the RNA world hypothesis (Kun et al., 2015), which suggests that all cells on primitive earth must have used RNA as its genetic material and DNA, being much more stable, would have replaced RNA as the genetic material eventually. However, there are also other hypotheses that suggest that it was the proteins that indeed formed first.
No matter which hypothesis, the underlying point in all of them is that intricate coordination between various molecules lead to the making of a fully functional, live cell.
Here I depict using acrylic art, the different organelles that exist within cells like the nucleus, mitochondria, chloroplast, endoplasmic reticulum, gogli apparatus, lysosome and ribosome. © Sunaina Rao.
NO! What you would see, would be a unit with several intricate, differently shaped compartments or rooms within it. How did this transformation happen?
As primitive cells that came into being on earth, started to evolve, some of them started to develop a membrane around their genetic material (Lusk and King, 2017), to provide it extra protection, and this compartment came to be called a nucleus (Pederson, 2010).
Some chlorophyll containing, green cells learnt to synthesise their own food using solar energy (photosynthesis), releasing oxygen into the atmosphere. On the arrival of oxygen, some cells learnt to use oxygen to derive energy. Some bigger cells took up some of these green, chlorophyll containing cells and started co-existing together (endosymbiosis). These chlorophyll containing compartments in the bigger cells (the present-day plant and plant like cells) came to be called the Chloroplast and the Oxygen harnessing compartments came to be called the Mitochondria.
Cells also started to evolve specific chambers called lysosomes (Sabatini and Adesnik, 2013), where they could digest the ingested food and recycle worn out components in the cell.
For the cell to make its own proteins, there needs to be orderly interaction between the RNA (the molecule that helps translate the genetic information in the DNA) and the amino acids (the subunits that make proteins). The cells hence started to evolve small molecules that could co-ordinate such interactions, called the ribosomes. Cells also evolved membranous channels, called the Endoplasmic Reticulum and Golgi Apparatus, that could help transport proteins to other parts of the cells and also to other cells6.
So, if you were to peep into your own skin cell, you would find all these chambers, called organelles (except the chloroplast, which is reserved for plant cells and some microorganisms only). Indeed, what you would find, is a fully functional city, with a well laid out infrastructure!
Here I depict using acrylic art, a fraction of the huge array of cells that make up organisms. Nerve cell in light pink, Protists in yellow, fungal cells in blue, plant cell in green and bacterial cells in dark pink. © Sunaina Rao.
Based on how cells started to evolve, it’s pretty clear that cells can exist is different shapes, sizes and forms and different organisms are indeed made of different kinds of cells.
Broadly speaking, cells can either be prokaryotic or eukaryotic. Prokaryotic cells are the simpler, smaller cells, where the genetic material is not enclosed by a membrane. They lack a nucleus and they also lack other complex organelles like the mitochondria and the endoplasmic reticulum and so on. So, they perform all their functions in a single room! Whereas, eukaryotic cells are the more developed cells with the nucleus and all the complex organelles. So, they have different rooms for different functions.
All the organisms that we find around us, broadly fall into three domains – Archaea, Bacteria and Eukarya (Woese et al., 2006). The Archaea and Bacteria includes most of the pathogenic and non-pathogenic organisms that we have heard of, like the bacteria that causes Cholera, Typhoid and Pneumonia and E-Coli, the bacteria that lives in our intestines. All these organisms are single celled and are prokaryotic.
Eukarya, on the other hand includes all organisms with eukaryotic cells. This would include some single celled organisms like the Amoeba and other multicellular organisms like Fungi, Plants and Animals.
Here I depict using acrylic art, the multicellularity in a plant. © Sunaina Rao.
Unicellular organisms, organisms that live as a single cell, reproduce and make more of its kind either sexually or asexually. All the resultant ‘daughter’ cells then become a separate life, which can go on to make more such life. However, during the evolutionary process, at some point, some of the cells after dividing, learnt to remain in groups, and evolved ways to remain glued together. Hence evolved multicellularity.
Multicellularity can be seen in many organisms belonging to the Eukarya domain, such as Fungi, animals, land plants and few other groups. Essentially, it provides the organism with a higher degree of structure, division of labour between cells and a well coordinated set up. If you look at your own body, you will find some 37.2 trillion cells, all coordinating activities with each other. Some covering the body, some carrying oxygen, some conducting electric impulses and some giving structure to the body, amongst many others. It functions more or less like a large company, with several departments!
Here I represent using acrylic art, an abstract illustration of what human breast cancer cells would appear like under a normal light microscope (left, in black and white) and how the same cells would appear under a fluorescence imager, if DNA was stained with Hoechst (blue) and the protein, Tubulin, was stained with a green fluorescence stain. © Sunaina Rao.
Modern day science, however, goes much beyond just observing organisms under the microscope. We are now able to extract cells from the body and grow it outside the body in conditions similar to that inside the body. We can stain the DNA and study its behaviour in different conditions. We can stain proteins in the cell and visualise their activity. We can manipulate DNA sequences and create our own protein. The possibilities are innumerable. All these studies have helped us understand the functioning of the cell better and thereby create better drugs and vaccines. But more than anything else, it has given us a better idea about the very essence of life and the beauty of its intricate design.