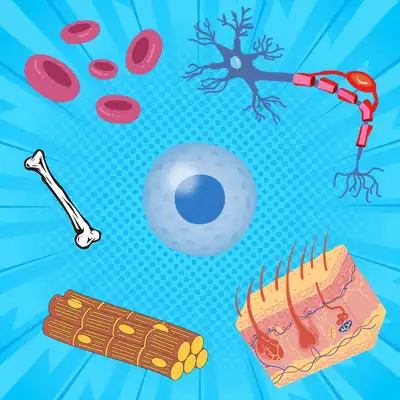
Everything you need to know about stem cells
What are stem cells, and what makes them so unique? What are the different types of stem cells, and how have they impacted modern medical science? Here is us answering all your questions about stem cells.
Ever observed a White Blood Cell (WBC) running after a microbe? If you have not, you should surely watch this fascinating video. What we see in the video is a neutrophil (type of WBC) relentlessly chasing a bacterial cell, twisting, and turning, pushing aside the adjacent Red Blood Cells (RBCs) until it finally engulfs the bacterium. Although what we see here, is a video shot in in vitro or laboratory conditions, it fairly replicates the neutrophil movement in the body.
During an infection, the infected cells as well as the pathogen, release certain chemoattractants – small protein molecules that can signal the circulating immune cells in the body. One of the important immune cells, popular for its engulfing or ‘phagocyting’ abilities is the neutrophil. On receiving a signal, the neutrophil flowing in the blood stream immediately gets polarised, forming a front and back end, and moves in the direction of the chemo-attractant. Eventually it exits the blood vessel and enters the tissue space, where it crawls holding on to the Extracellular Matrix (ECM) which is largely composed of fibrous proteins. Finally, on encountering the infected cell or the pathogen, it initiates phagocytosis (Michael and Vermeren, 2019). But how does the neutrophil actually bring about such coordinated movement? Let us find out!
Representation of the actin cytoskeleton network in a neutrophil. Orange - Cell with its cell body (consisting of the nucleus and cellular organelles); Green - Actin cytoskeleton; Blue - Focal adhesions holding on to the ECM; Red - ECM/Substrate; Yellow - Chemoattractant molecules released by the microbe. © Sunaina Rao.
To understand how a cell moves, we need to first look into its cytoskeleton. Just like the human body has a skeletal system, the cell too is supported by its own skeletal network composed of protein filaments, that not only help the cell move, but also gives it shape and polarity. One such filament network is the actin microfilament.
To give you a brief idea of its structure, the actin microfilament network is made of ‘G-actin’ monomers that polymerise in the presence of Adenosine Tri-Phosphate (ATP) and Mg+2 ions to form long filamentous ‘F-actin’. These long filaments further organise themselves into helical structures, with each filament wound around the other. The polymerising end is denoted as the ‘plus’ end. Now at the other end of the filament, denoted as the ‘minus’ end, G-actin monomers are disassembled and are recycled to the growing end, where they can again partake in polymerisation. This kind of continuous polymerisation and depolymerisation makes the actin microfilament a highly dynamic structure.
In the cell body these filaments are arranged as a 3D network. Whereas just underneath the membrane, they form a 2D network, much like a spider’s web. They bind to the membrane via membrane proteins, one of them being the integrins. The integrins in turn adhere to the ECM proteins outside the cell to form stable focal adhesions. This system hence connects the inside of the cell to the outside, enabling the cell to achieve a tight grip on its substrate.
The cell forms a frontward protrusion (represented by the region above the dotted line), towards the chemoattractant gradient. © Sunaina Rao.
So how does the neutrophil know the direction of its movement? Much like we are drawn towards a delicious smell, the neutrophil literally ‘smells’ the chemoattractant. The chemoattractant molecules activate certain cell signalling protein receptors on the cell membrane (called GPCRs – G Protein Coupled Receptors) which generate a signalling cascade inside the cell. The end that receives the signal automatically becomes the front end, and this end steers the cell in the direction of the chemoattractant gradient. The signal activation at the front end, initiates polymerisation of G-actin there, forming F actin. This polymerisation pushes against the cell membrane, thereby making a protrusion called the ‘lamellipodium’ (Tsai et al., 2019, Schaks et al., 2019).
The cell assembles fresh focal adhesions at the front end (represented in blue) and disassembles the focal adhesions at the back. © Sunaina Rao.
To prevent the extended membrane from retracting back, the cell assembles fresh focal adhesions across the protrusion on the ventral (lower) side, allowing the cell to clasp the substrate (in this case the ECM) firmly at the front end. On the other hand, the focal adhesions at the rear portion of the cell is disassembled, thereby loosening the grip on the substrate there.
Membrane tension combined with the action of myosin (in red), pushes the entire cell body forward. © Sunaina Rao.
The extension of the membrane at the front, creates a tension across the cell membrane, causing the membrane at the back of the cell to retract. Additionally, another very important protein molecule comes into play at this point, and that is Myosin. Myosin in a crucial motor protein that remains active at the rear end of the cell. It clings on to the actin filaments at the back and exerts contractile forces on them. This creates a hydrodynamic push, that propels the entire cell body frontward (Ananthakrishnan and Ehrlicher, 2012).
The neutrophil repeats this motion, turning and changing direction if need be, until it reaches and engulfs the microorganism. Understanding cell mobility has been an important area of research for years as it could give us essential insights into immune function, wound repair, tissue regeneration, and the pathology of diseases like cancer, all of which are dependent on cell mobility. It might seem amazing how such a tiny, microscopic cell can put together this highly coordinated act in order to move itself. But indeed, this is a result of billions of years of evolution!